PhD thesis: Potential of the mosquito Aedes malayensis as an arbovirus vector in South East Asia
Survey of the sylvatic mosquito fauna in a forested area of the Nakai Nam Theun National Protected Area
Supervisors
Dr. Paul Brey (IPL)
Dr. Louis Lambrechts (Institut Pasteur / CNRS 2000)
PhD Student
Elliott Miot
Background
The Lao mosquito fauna is still not well characterized, especially in remote forested areas such as our study area. The work of Rueda et al. [1] began to establish a comprehensive checklist of mosquito species in the country, but it was mainly based on a literature review and only a few field collections.
Methods
In order to update the checklist of mosquitoes in our study area, we performed larval and adult mosquito collections in the NNT NPA along several rivers: Nam Theun (January 2012), Nam Mon (March 2012), Nam Noy (December 2011, March-April 2012, February, March, May, August, November, December 2017, and February-March 2018), and Nam On (May 2017) (Figure 1).
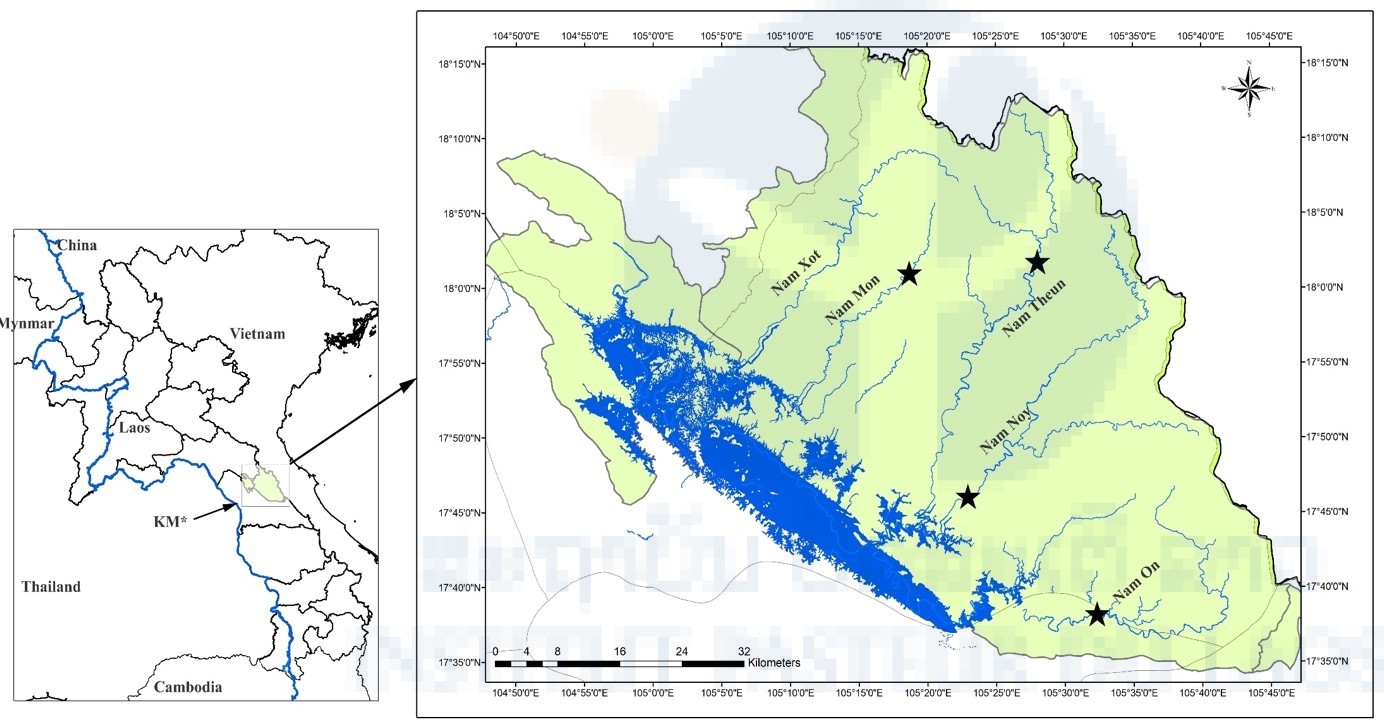
Figure 1. Map showing sampling locations of mosquitoes in Lao PDR. KM = Khammuane Province; Black stars = sampling locations; Blue line = Mekong River and its tributaries.
Mosquito larvae were collected in various types of breeding sites such as rock pools along the riverbanks, tree holes, temporary pools of water, fruit husks, and bamboo shoots. We also constructed bamboo traps, i.e. large bamboos cut in small pieces and filled with freshwater, that we deployed across the Nam Noy field site to create semi-natural breeding sites. Adult mosquitoes were captured using a combination of commercial traps (e.g. CDC light traps, BG sentinel traps) and active collections using vacuum-powered and mouth aspirators, and butterfly nets. Larvae were reared until adulthood and sorted morphologically back in the laboratory.
Results
During our field surveys, we identified 54 mosquito taxa belonging to 11 genera, of which 15 species were new records in Laos. We also described Ae. malayensis, a species found throughout South East Asia [2-4], for the first time in Laos based on morphological characters and molecular analyses. We produced photos of the morphological characters allowing proper identification of Ae. malayensis and distinction from closely related species that are found in sympatry, such as Ae. albopictus (Figure 2 & 3). We also provided information on the bionomics of Ae. malayensis.
Figure 2: Morphological comparison of the thorax. (A) Ae. malayensis – a supraalar area of thorax with a patch of pale scales extended upward toward the scutellum. (B) Ae. albopictus – a supraalar area of thorax with a spot of pale scales not extended toward the scutellum.
Figure 3: Morphological comparison of the abdominal terga IV-VI. (A) Ae. malayensis – dorsal white bands connected to lateral pale patches. (B) Ae. albopictus – dorsal white bands separated from lateral spots.
Successful colonies of Ae. albopictus and Ae. malayensis were derived from the collections, which allowed subsequent laboratory studies on these sylvatic mosquito populations.
Publications
Motoki MT, Vongphayloth K, Rueda LM, Miot EF, Hiscox A, Hertz JC, and Brey PT. New records and updated checklist of mosquitoes (Diptera: Culicidae) from Lao People’s Democratic Republic, with special emphasis on adult and larval surveillance in Khammuane Province. J Vector Ecol. 2019;44: 76–88. doi:10.1111/jvec.12331
Motoki MT, Miot EF, Rueda LM, Vongphayloth K, Phommavanh N, Lakeomany K, Debboun M, Hertz JC, and Brey PT. First Record of Aedes (Stegomyia) malayensis Colless (Diptera: Culicidae) in the Lao People’s Democratic Republic, Based on Morphological Diagnosis and Molecular Analysis. US Army Med Dep J. 2018: 1–7.
Identification of sylvatic mosquito species attracted to humans in a forested area of the Nakai Nam Theun National Protected Area
Background
Little is known about the vector status, ecology, and behavior of mosquito species in Laos. In addition to vector competence, vectorial capacity strongly depends on mosquito ecology and feeding behavior.
Host preference, host availability, vector abundance, feeding frequency, encounter rate, and the temporal pattern of blood-feeding activity are some of the main ecological parameters that determine the effective contact between vectors and hosts [5]. Thus, characterizing the abundance, richness, diversity, and biting behaviors of mosquito species is an essential step in order to assess the risk of spillover of zoonotic, vector-borne pathogens to the human population [6]. The same is true for assessing the risk of spillback of human vector-borne pathogens establishing novel sylvatic transmission cycles in regions where they were previously absent [7,8].
Methods
We conducted a total of four 1-week missions in August (rainy season), November-December (dry season) 2017 and twice in March 2018 (intermediate season) in the NNT NPA. Mosquito species attracted to humans were specifically targeted using human-baited double-net traps [9] (Figure 4) deployed in three habitats (riverside, low-cover forest, high-cover forest) along a trail going from the Nam Noy riverbank to deeper in the forest (Figure 5). Mosquitoes were captured around the clock, sacrificed, grouped per 1-hour interval, and identified morphologically back in the laboratory. Based on the captures, we evaluated the abundance, richness, diversity, and daily activity pattern of human-attracted mosquito species and compared these indices between habitats and seasons.
- Figure 4: Human-baited double-net traps in situ.
- Figure 5: Map of the Nam Noy field site with the location of the collection sites. Generated using ©Google Maps. Purple, red and yellow marks show the collection locations in the riverside habitat, low-cover forest habitat, and high-cover forest habitat, respectively.
Results
We collected a total of 1,018 females that were morphologically assigned to 9 genera and 26 mosquito taxa, of which 87.6% represented only 8 mosquito species (Figure 6). Some of them are known or putative arbovirus vectors such as Ae. albopictus, Cx. vishnui, Ar. subalbatus, and Ae. malayensis. Others have been poorly studied and little is known about their potential as pathogen vectors such as Heizmannia species, Ae. desmotes, and Ar. jugraensis.
Figure 6: Species abundance of human-attracted mosquitoes stratified by season and habitat. Heat map of the log10- transformed a total number of mosquitoes caught in the human- baited traps shown by season (rainy, dry, intermediate) and habitat (riverside, low-cover forest, high-cover forest) for each species. Abundance ranges from dark blue (low) to red (high). Analyses of species richness and diversity revealed that they were significantly higher during the rainy season, especially in the low-cover forest habitat (Figure 7 & 8)
Figure 7: Species richness of human-attracted mosquitoes stratified by season and habitat. Boxplots of the daily number of mosquitoes caught in the human-baited traps shown by season (rainy, dry, intermediate) and habitat (riverside, low-cover forest, high-cover forest). Each point in the boxplots represents the species richness of a 24-hour mosquito collection period. Letters above the boxplots indicate the statistical significance of the differences. Conditions sharing a letter are not significantly different.
Figure 8: Diversity of human-attracted mosquitoes stratified by season and habitat. Boxplots of the daily Shannon diversity (H’) index of mosquitoes caught in the human-baited traps by season (rainy, dry, intermediate) and habitat (riverside, low-cover forest, high-cover forest). Each point in the boxplots represents the H’ index of a 24-hour mosquito collection period. Letters above the boxplots indicate the statistical significance of the differences. Conditions sharing a letter are not significantly different.
Daily activity patterns showed that all human-attracted mosquito species collected were only active during daytime regardless of seasons or habitats (Figure 9). Host-seeking activity differed between the low-cover forest habitat, where mosquitoes were most active during the afternoon, and the high-cover forest habitat, where they were more active in the morning, regardless of the season.
Figure 9: Daily pattern of human-seeking mosquito activity stratified by habitat and season. The mean hourly number of mosquitoes caught in the human-baited traps is plotted over time by habitat (riverside, low-cover forest, high-cover forest) and season (rainy, dry, intermediate). Hourly data were collected for 24-hour periods (rainy season: 6 consecutive days and nights; dry season: 6 consecutive days and nights; intermediate season: 2 x 6 consecutive days and nights).
The results of this study improve our understanding of mosquito-human interactions in the NNT NPA by providing new information on the species abundance, richness, diversity, and daily activity pattern of mosquitoes caught in human-baited traps. We characterized several human-attracted mosquito species, including Ae. malayensis, in the primary forest of Laos, which paves to way for identification of potential bridge vectors in this area.
Publication
Miot EF, Motoki MT, Phommavanh N, Lakeomany K, Vongphayloth K, Hertz JC, Lambrechts L, and Brey PT. Diversity, abundance and daily activity pattern of human-seeking mosquitoes in a forested area of the Nakai district, Laos. Manuscript in preparation.
Potential of the sylvatic mosquito Aedes malayensis to act as an arbovirus bridge vector in a forested area of the Nakai Nam Theun National Protected Area
Background
Following our initial field surveys, we chose to focus on the mosquito Ae. malayensis to further characterize its potential to act as an arbovirus bridge vector in the NNT NPA. This was done by evaluating its vector competence and its attraction to human scent in laboratory conditions.
Methods
We measured the vector competence of our laboratory colony of Ae. malayensis recently derived from a sylvatic population (see Chapter 1) for DENV and YFV, using an urban Ae. aegypti population from Laos as a positive control. Mosquito was orally challenged with a low passage DENV-1 isolate from Laos and a low-passage YFV isolate belonging to the West African lineage. Additionally, we performed behavioral experiments using a dual-port olfactometer to assess the specific attraction of this Ae. malayensis population to human odor in the presence of CO2 (Figure 12A).
Results
We found that our sylvatic Ae. malayensis population was competent for DENV-1, but to a lesser extent than our Ae. aegypti control (Figure 10). Due to low YFV infection rates overall, we were not able to conclusively demonstrate that this Ae. malayensis population was competent for YFV, but it was likely less competent than our Ae. aegypti control (Figure 11).
Figure 10: Vector competence of sylvatic Ae. malayensis and Ae. aegypti controls after exposure to 1.38 x 107 FFUs/ml of DENV-1. Bars represent the percentage of virus-positive mosquitoes 14 days post-infectious blood meal and the error bars are the 95% confidence intervals of the percentages. The infection rate (IR) is the proportion of blood-fed females with an infected body. Dissemination rate (DR) is the proportion of infected females with virus disseminated to the head tissues. Transmission rate (TR) is the proportion of females with a disseminated infection that shed virus in their saliva. Transmission efficiency (TE) is the overall proportion of blood-fed females that shed virus in their saliva. The Ae. aegypti population was included as a positive control. The figure compiles data from two independent experiments that did not differ significantly. **p < 0.01; ***p < 0.001. Publication
Figure 11: Vector competence of sylvatic Ae. malayensis and Ae. aegypti controls after exposure to 1.84 x 106 FFUs/ml of YFV. Bars represent the percentage of virus-positive mosquitoes 14 days post-infectious blood meal and the error bars are the 95% confidence intervals of the percentages. The infection rate (IR) is the proportion of blood-fed females with an infected body. Dissemination rate (DR) is the proportion of infected females with virus disseminated to the head tissues. Transmission rate (TR) is the proportion of females with a disseminated infection that shed virus in their saliva. Transmission efficiency (TE) is the overall proportion of blood-fed females that shed virus in their saliva. The Ae. aegypti population was included as a positive control. ***p < 0.001.
The olfactometer bioassays allowed us to measure both flight activity (i.e. the percentage of females that spontaneously left the release chamber) and specific attraction to human odor (i.e. the percentage of trapped mosquitoes that chose the trap with human odor). We observed that in the presence of human odor combined with CO2, a higher proportion of Ae. malayensis females were activated and started flying compared to CO2 alone (Figure 12B). However, there was no specific attraction to human odor, with equal proportions of mosquitoes caught in the trap with human odor combined with CO2 and in the trap with CO2 alone (Figure 12C).
Figure 12: Lack of laboratory evidence for Ae. malayensis attraction to human odor. (A) Schematic and pictures of the experimental setup to measure attraction to human odor in a dual-port olfactometer. (B) Flight activity is the percentage of female mosquitoes that exited the release chamber after 20 min. (C) Attraction to human odor was estimated as the percentage of trapped mosquitoes that chose the trap with human odor, which ranges from 0% (full attraction to CO2 without human odor) to 100% (full attraction to CO2 with human odor). The red, vertical dashed lines indicate the expected percentage of trapped mosquitoes when there is no preference for either trap (50%). Error bars represent 95% confidence intervals of the percentages. Letters next to or above the bars indicate statistical significance. Conditions with a letter in common are not significantly different from each other. The data are shown in (B) and (C) are pooled from 6 and 9 separate replicate trials for CO2 only vs. CO2 only and CO2 + Human odor vs. CO2 only designs, respectively, which did not differ significantly. The Ae. aegypti population was included as a positive control.
The relatively modest vector competence for DENV and YFV, combined with a lack of detectable attraction to human odor in laboratory conditions, indicate a low potential for this sylvatic population of Ae. malayensis to act as an arbovirus bridge vector in our study area. Nevertheless, we caution that opportunistic feeding on humans (see Chapter 2) may occasionally contribute to bridging sylvatic and human transmission cycles. Further assessment of the risk of DENV and/or YFV spillback associated with Ae. malayensis should account for additional risk factors such as the presence of susceptible non-human primates and the probability of human-mediated introduction of arboviruses into the area.
Publication
Miot EF, Calvez E, Aubry F, Dabo F, Grandadam M, Marcombe S, Oke C, Logan JG, Brey PT, and Lambrechts L. Potential of the sylvatic mosquito Aedes malayensis to act as an arbovirus bridge vector in a forested area of the Nakai district, Laos. Manuscript under review for publication in Parasites & Vectors.
Potential of peridomestic Aedes malayensis mosquitoes to transmit yellow fever virus in Singapore
Background
Following our evaluation of the vectorial capacity of a sylvatic population of Ae. malayensis in Laos (see Chapter 3), we extended our investigation to a peridomestic Ae. malayensis population found in urban parks of Singapore.
This Ae. malayensis population was previously colonized by our collaborators at Duke-NUS Medical School who suspected that it could contribute to “cryptic” arbovirus transmission. Although vector control measures in Singapore achieve very low densities of Aedes mosquitoes [10], arboviruses such as DENV, CHIKV, and ZIKV have re-emerged in Singapore in the last two decades [11-14]. Indeed, this peridomestic Ae. malayensis population was recently shown to be experimentally competent for DENV and CHIKV [15]. However, its vector competence for YFV had not been evaluated, and its propensity to bite humans was unknown.
Until now, the Asia-Pacific region has remained YFVfree. However, the increasing influx of travelers coming from endemic regions of Africa and South America where recent YFV outbreaks have occurred has significantly increased the risk of YFV introduction in places like Singapore, a major hub for trade and tourism [16,17]. YFV introduction into the Asia-Pacific region was confirmed in 2016 when 11 workers infected with YFV in Angola returned to China, although fortunately, it did not subsequently result in local transmission of the virus [18].
Methods
To evaluate the ability of Ae. malayensis to contribute to YFV transmission in Singapore, we used the colony established in 2014 by our collaborators in Singapore. First, we measured the vector competence of this Ae. malayensis colony for YFV, using Ae. aegypti as a positive control. Mosquito was orally challenged with a low-passage YFV isolate belonging to the West African lineage. Second, I performed a small-scale field survey in several parks and a forested area of Singapore in March 2019 to evaluate the probability of host-vector contact between humans and Ae. malayensis. I sampled mosquitoes at 6 locations from 7 a.m. to 7 p.m. using a human-baited double-net trap: East Coast Park zone A (2 days), East Coast Park zone C (1 day), Mount Faber Park (1 day), Clementi woods park (1 day), West Coast Park (1 day), and a forested area in the northern part of the city in Sembawang (3 days). We also obtained data from the island-wide Gravitrap surveillance network implemented by the Singapore National Environment Agency (NEA) to monitor the spatial and temporal variability of adult Aedes mosquito populations.
Results
Overall, the peridomestic Ae. malayensis population from Singapore was experimentally competent for YFV to a similar level as our Ae. aegypti control (Figure 13 &14).
Figure 13: Peridomestic Ae. malayensis in Singapore are orally susceptible to YFV. Dose-response curves are shown for the Ae. malayensis population from Singapore understudy and a control Ae. aegypti population. The infection rate is the proportion of blood-fed females testing YFV-positive 10–14 days post blood meal. Dissemination rate is the proportion of YFV-infected females with YFV-positive wings/legs or heads 14 days post blood meal. The line represents the logistic regression of the data and the shaded area is the 95% confidence interval of the fit. The data shown are combined from two separate experiments.
During the small-scale field survey, Ae. malayensis was caught in the human-baited trap in one of the urban parks (Sembawang). The most abundant species collected was Ae. albopictus (89%) followed by Ae. malayensis (9%). Moreover, out of the 504,771 mosquitoes collected in Gravitraps, the predominant species was Ae. aegypti (80%), but a total of 1,931 Ae. malayensis were caught in 1,741 traps (Figure 15).
Figure 15: Distribution of Aedes malayensis in highrise apartment blocks in Singapore. Map based on data collected from the island-wide Gravitrap surveillance network in 2018.
Together, this study provided evidence that peridomestic Ae. malayensis mosquitoes in Singapore are competent vectors for YFV, which may not only engage in contact with humans in urban parks but also breed in domestic settings such as housing blocks. Therefore, we conclude that Ae. malayensis could contribute to YFV transmission in Singapore if the virus were to be introduced.
Publication
Miot EF, Aubry F, Dabo S, Mendenhall IH, Marcombe S, Tan CH, et al. A peridomestic Aedes malayensis population in Singapore can transmit yellow fever virus. PLoS Negl Trop Dis. 2019;13: e0007783. doi:10.1371/journal.pntd.0007783
References
1. Rueda LM, Vongphayloth K, Pecor JE, Sutherland IW, Hii J, Debboun M, et al. Mosquito Fauna of Lao People’s Democratic Republic, with Special Emphasis on the Adult and Larval Surveillance at Nakai District, Khammuane Province. US Army Med Dep J. 2015;: 25–32.
2. Huang Y-M. Contributions to the Mosquito Fauna of Southeast Asia. XIV. The Subgenus Stegomyia of Aedes in Southeast Asia I – The Scutellaris Group of Species. Contr Am Entomol Inst. 1972;: 1–109.
3. Tewari SC, Hiriyan J, Reuben R. Epidemiology of subperiodic Wuchereria bancrofti infection in the Nicobar Islands, India. Trans R Soc Trop Med Hyg. 1995;89: 163–166.
4. Rattanarithikul R, Harbach RE, Harrison BA, Panthusiri P, Coleman RE, Richardson JH. Illustrated keys to the mosquitoes of Thailand. VI. Tribe Aedini. Southeast Asian J Trop Med Public Health. 2010;41 Suppl 1: 1–225.
5. Kramer LD, Ebel GD. Dynamics of flavivirus infection in mosquitoes. Adv Virus Res. 2003;60: 187–232.
6. Vasilakis N, Cardosa J, Hanley KA, Holmes EC, Weaver SC. Fever from the forest: prospects for the continued emergence of sylvatic dengue virus and its impact on public health. Nat Rev Microbiol. 2011;9: 532–541. doi:10.1038/nrmicro2595
7. Bryant JE, Holmes EC, Barrett ADT. Out of Africa: a molecular perspective on the introduction of yellow fever virus into the Americas. PLoS Pathog. 2007;3: e75. doi:10.1371/journal.ppat.0030075
8. Tangena J-AA, Thammavong P, Hiscox A, Lindsay SW, Brey PT. The Human-Baited Double Net Trap: An Alternative to Human Landing Catches for Collecting Outdoor Biting Mosquitoes in Lao PDR. Hansen IA, editor. PLOS ONE. Public Library of Science; 2015;10: e0138735. doi:10.1371/journal.pone.0138735
9. Egger JR, Ooi EE, Kelly DW, Woolhouse ME, Davies CR, Coleman PG. Reconstructing historical changes in the force of infection of dengue fever in Singapore: implications for surveillance and control. Bull World Health Organ. World Health Organization; 2008;86: 187–196. doi:10.2471/ BLT.07.040170
10. Hapuarachchi HC, Koo C, Rajarethinam J, Chong C-S, Lin C, Yap G, et al. Epidemic resurgence of dengue fever in Singapore in 2013-2014: A virological and entomological perspective. BMC Infect Dis. BioMed Central; 2016;16: 300. doi:10.1186/s12879-016-1606-z
11. Koh BKW, Ng L-C, Kita Y, Tang CS, Ang LW, Wong KY, et al. The 2005 dengue epidemic in Singapore: epidemiology, prevention and control. Ann Acad Med Singap. 2008;37: 538– 545.
12. Leo YS, Chow ALP, Tan LK, Lye DC, Lin L, Ng LC. Chikungunya outbreak, Singapore, 2008. Emerging Infect Dis. 2009;15: 836–837. doi:10.3201/eid1505.081390
13. Maurer-Stroh S, Mak T-M, Ng Y-K, Phuah S-P, Huber RG, Marzinek JK, et al. South-east Asian Zika virus strain linked to cluster of cases in Singapore, August 2016. Euro Surveill. European Centre for Disease Prevention and Control; 2016;21: 1232. doi:10.2807/1560-7917.ES.2016.21.38.30347
14. Mendenhall IH, Manuel M, Moorthy M, Lee TTM, Low DHW, Missé D, et al. Peridomestic Aedes malayensis and Aedes albopictus are capable vectors of arboviruses in cities. Apperson C, editor. PLoS Negl Trop Dis. 2017;11: e0005667. doi:10.1371/journal.pntd.0005667
15. Gubler DJ. Pandemic yellow fever: a potential threat to global health via travelers. J Travel Med. 2018;25: 160. doi:10.1093/jtm/tay097
16. Butler D. Fears rise over yellow fever’s next move. Nature. 2016.: 155–156. doi:10.1038/532155a
17. World Health Organization (WHO). Emergencies preparedness, response: Yellow Fever – China. In: WHO [Internet]. World Health Organization; 22 Apr 2016 [cited 3 Apr 2019]. Available: http://www.who.int/csr/don/22-april-2016-yellow-fever-china/en/