Risk Of Vector-Borne Diseases In Relation To Rubber Plantations In Lao PDR
Project leader: Dr Paul Brey
Member of staff: Julie-Anne Tangena, Phoutmany Thammavong, Somsanith Chonephetsarath, Honglakhone Xayasing
ECOnomic development, ECOsystem MOdifications, and emerging infectious diseases Risk Evaluation
Background
South-East Asia (SEA) is experiencing unprecedented economic growth which is transforming land-use on a massive scale in the region. An increasing number of studies show that these land use changes are a major force behind the surge of emerging infectious diseases. In recent years epidemics of SARS, Nipah virus, cholera, malaria, dengue and Japanese encephalitis have been documented [1-4]. Vector-borne diseases are emerging and increasing in areas where ecological disruption occurs, driven by population growth, land use change, urbanization, agricultural reforming, and human migration [5-9].
China is at the heart of the economic growth in the region and its economy has been growing since 1978 at a rate of ± 9 % per year, approximately three times the global average [10]. The economy of the region has been growing since the major economic reforms, with gross domestic product per capita increasing from 1,339 USD in 1978 to 10,540 USD in 2010 [11]. China officially became the second largest economy in February 2011 and it is likely to surpass the United States to become the largest economy in 2020 [12].
All SEA countries are benefitting from the Chinese growth [13, 14]. China imported 341 billion US dollars’ worth of products from SEA in 2009, which was nearly half of China’s total import [13].
One of the clear examples of the strong economic ties between China and SEA is the rubber industry which supports the rapidly expanding Chinese car industry. In China 18.5 million cars were manufactured in 2010 for which an estimated 3.85 million tons (34 % of the global demand) of rubber was needed, mainly for tyres [15, 16]. Rubber consumption has been increasing 4% annually in the last decades with 42% of this being produced naturally from plantations [15, 17]. Natural rubber production could not keep up with the increased demand and caused prices to reach record highs in 2011 after the natural rubber stock of the world reached a negative 367,000 tonnes in 2010 [18-21]. In Lao PDR this meant latex prices increased from 3,000 kip/kg (0.37 USD) in 2002 to an inflated 15,000 kip/kg (1.87 USD) in 2011 [22]. The increase in price of natural rubber from 2002 onwards encouraged many more to invest in rubber plantations. In Viet Nam the total area for rubber plantation rose from 77,000 ha in 1976 to 465,000 ha in 2005, with land designated for rubber plantations close to 700,000 ha [23]. Presently an estimated 9.161 million ha of rubber plantations exists in SEA [24]. Rubber plantations are largely found in Thailand, Indonesia and Malaysia, together accounting for 72% of global natural rubber production. Thailand in 2009 had 2.8 million ha of rubber growing region with almost 5 million tonnes of natural rubber produced every year [25, 26]. South East Asia supplies 97 % of the world’s natural rubber [27].
The high natural rubber production after the peak of investment together with the global recession from 2008-2010 created an excess of natural rubber and natural rubber prices have slumped since 2011 [28, 29]. In December 2013 natural rubber production growth was 3% whilst it’s utilization shrank [30]. Natural rubber prices in Lao PDR dropped from 15,000 kip (1.87 USD) in 2011 to 7,000-8,000 kip (0.75-0.87 USD) per kg in 2014 [22]. In 2013 the world natural rubber production was 12,042,000 tonnes with 687,000 tonnes of rubber overproduced, resulting in 3,006,000 tonnes in stock [31]. Consequently from the beginning of 2014 natural rubber prices have dropped more than 20% [32, 33]. The decline in the price of rubber is concerning many natural rubber producers and have even led to protests in Thailand [34, 35]. Although difficult to predict, the International Rubber study Group expects demand for natural rubber to increase until at least 2020 [15, 21].
It takes about five to eight years for the trees to mature, from when the trees can be tapped for rubber for up to 30 years. The whitish latex used for rubber production is present outside the phloem in latex vessels of the bark. These vessels are curved at a 30o angle up the tree in a right-handed spiral. This spiral makes tapping latex very difficult and requires a certain skill. A sequence of thin slices of bark are cut without damaging the growing layer (Figure 1). Every worker is able to tap between 300-450 trees per night equivalent to 1ha of rubber plantation.
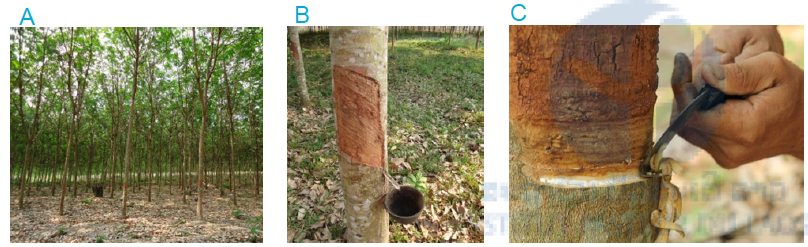
Figure 1: Rubber plantation in Luang Prabang province, Lao PDR (A) Rubber plantation (B) Rubber tree with collecting cup (C) Cutting of rubber tree for tapping
Lao PDR has seen a high increase in rubber plantations (Table 1), where relatively low number of plantations are present compared to neighbouring countries [17, 27]. This is a new kind of mass farming not seen in Lao before. There are two different kinds of rubber plantations in Lao PDR; small scale Lao owned rubber plantations and industrial scale plantations. After 2015 the area of rubber plantations will continue growing with currently 342,400ha of land designated for rubber plantations[36].
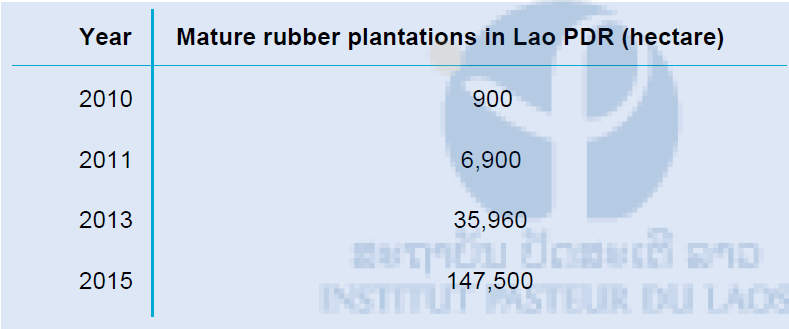
Table 1: Mature rubber plantations in Lao PDR (hectare)
In the next decade an estimated 6 million people will be working as tappers on rubber plantations in SEA, which highlights the importance of understanding the exposure risk to mosquito-borne diseases for these workers. Currently there is a paucity of information on vector-borne disease risk in rubber plantations despite the fact malaria epidemics have been linked to rubber plantations in SEA for over a century, as have outbreaks of dengue and the presence of lymphatic filariasis [37-41]. Data on mosquitoes, vector-borne disease epidemiology and human movement are often difficult to collect for rubber plantation areas with low quality surveillance, unregistered migration, insufficient capacity and difficult to reach areas.
The range of vectors found in rubber plantations is likely to be broad and similar throughout Asia. In one study in India vectors of dengue, chikungunya, Japanese encephalitis, malaria and filariasis were found in rubber plantations [42]. Rubber plantations are unnatural forests with generally higher humidity and lower temperatures than other non-tree crops. This is a habitat that some important vector species like Anopheles dirus and Aedes albopictus prefer [40, 43-46]. These extremely anthropophilic mosquitoes thrive in these unnatural forested areas of SEA as there are sufficient larval breeding sites, high humidity, stable temperatures, high rainfall and frequent contact with primates and people.
Seasonal workers at the plantations could create a whole new dynamic to the vector-borne diseases in the plantations. Currently 37,500 people work on rubber plantations in Lao PDR of which 95.4% are Lao [36]. The increase in rubber plantations in the coming decade is expected to create work for another 177,700 people. This will increase the need for seasonal workers from other areas. These temporary workers may not have immunity against the local diseases and are more likely to develop serious adverse effects. Additionally, these workers can spread the disease when they travel to areas where the vectors are already established. Even more worrying is the possibility to introduce a drug resistant strain, like ACT resistance, in an area by either returning home with a resistant strain or transporting the resistant strain to the place of work. It is suggested that the presence of high numbers of different vector mosquitoes combined with the increase in seasonal rubber workers and their high exposure to the vector mosquitoes is creating a ‘perfect storm’ in and around rubber plantations for future disease outbreaks
Rationale
The area of land cultivated for rubber is expanding rapidly in Lao PDR. We anticipate that the changes in ecology from primary and secondary rainforest, to rubber cultivation and the maturation of these rubber trees is likely to result in an altered risk from vector-borne diseases; predominantly malaria, dengue and chikungunya. It is envisaged that this study will provide an opportunity to understand the vector ecology in rubber plantations and be able to advise organizations on how to decrease vector-borne disease incidence. This study will be of relevance to public health workers, governments and those working in the rubber industries of Lao PDR and other countries in SEA.
All methods were approved by the Lao Ethics committee and Durham University ethics committee. The comparison study was also discussed with the CORC-ethics committee of Institut Pasteur and approved.
Overall goal: To assess the potential risk of vector-borne disease infections arising in rubber plantations
+ Recognize possible vectors and the diseases they carry in the rubber plantations
+ Identify at which times rubber workers are exposed to vectors
+Write public health advice for health workers, governments, and those working in the rubber industries of Lao PDR and other countries in South-East Asia how to decrease and/or keep vector-borne disease incidence low
Methods
During 2015 the ECOMORE team has been busy conducting the final fieldwork and analysing the results of previously conducted fieldwork. We have been focussing on the publication of our results in scientific journals, presenting our work at different national and international conferences, sharing our results and recommendations with stakeholders. Most importantly we have been working closely with the Lao government to include our recommendations in policy.
Longitudinal study on mosquito ecology in rubber plantation
To understand the ecology of the mosquitoes in the rubber plantations, we compared the mosquito number and diversity of the plantation with the forest and village for one year. We collected mosquitoes in the different habitats using the human-baited double net trap (Figure 2), which does not expose participants to mosquitoes. In 2013-2014 mosquitoes were collected in three study areas located in bordering Nane and Xieng Ngeun district (Luang Prabang province) where the following four habitats were present; immature rubber plantation, mature rubber plantation, village and forest. In 2013 we sampled mosquitoes with three participants every hour for two days and two nights in the three study areas every month from July to November. In 2014 this was carried out every two months from January to July. A total of 3,888 hours of data was collected in each habitat during the collection period of 2013-2014.
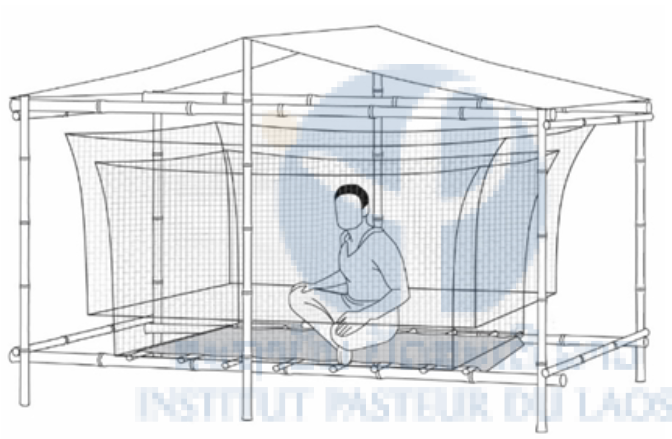
Figure 2: The Human-baited Double Net trap method
Vectorial capacity
To understand the importance of different vector species in the transmission of disease often the vectorial capacity is calculated. This vectorial capacity (R0) is the number of malaria/dengue cases derived from one infective case before he/she dies or is cured. If the R0 is lower than one, the disease goes extinct. However if the R0 is larger than one the disease will be able to establish itself in the area.
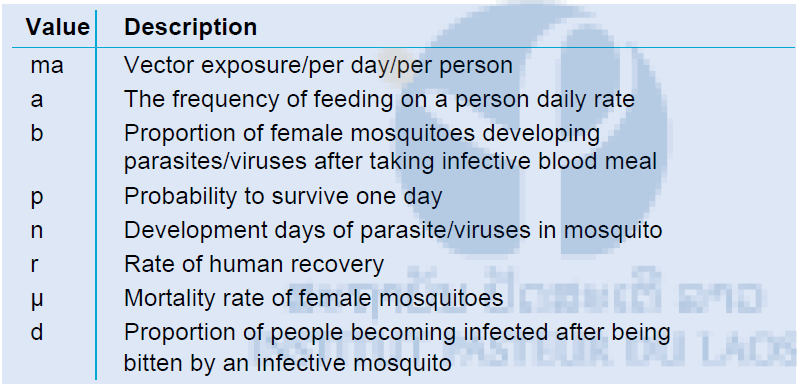
Table 2: Value of formula and description
To calculate this capacity we needed to conduct an additional study in the field to identify the parity rate of malaria and dengue vectors in the field. In June and July 2015 we collect Anopheles and Aedes albopictus mosquitoes for 42 nights using the human-baited double net traps in the village, secondary forest, immature and mature rubber plantation of village Thinkeo. Mosquito were collected by two participants in each habitat for 6 hours during the highest collection times of malaria vectors according to previous fieldwork in the area, from 17:00 to 23:00.
After identification, the abdominal condition of all female Anopheles and Aedes albopictus mosquitoes were noted in the laboratory form and grouped as unfed, freshly fed, half-gravid, and gravid. All unfed and freshly fed Anopheles and Aedes albopictus mosquitoes were dissected and parity was determined using the Detinova method [47]. Females in which the ovaries have coiled tracheolar skeins are nulliparous and have not taken a blood meal yet not have laid eggs (Figure 3A). Ovaries in which the tracheoles have become stretched out are parous and have laid eggs at least once (Figure 3B).
Figure 3: Ovaries dissected from female Ae. albopictus; A) nulliparous mosquito with coiled tracheolar skeins B) parous mosquitoes with stretched out tracheolar skeins
Larval survey
To understand where mosquitoes breed in the rubber plantations, a larval survey was conducted from August 2014 until December 2014 on a monthly basis using a systematic approach. Surveys were done in immature rubber plantations, mature rubber plantations and villages. In the mature rubber plantations random collecting cups were checked and all possible breeding sites were analysed. Data on water quality of all identified water bodies, their size, turbidity, location, presence of mosquito larvae and other insects were noted. Mosquito larvae were collected and reared at the field laboratory for identification purpose. Surveys were conducted monthly with the help of two villagers (Figure 4).
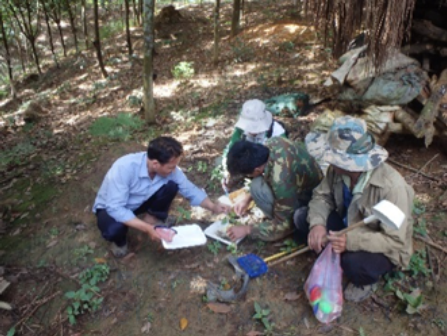
Figure 4: Larval survey conducted by our team and local villagers
Molecular data, malaria vector species identification
The Anopheles complexes collected during the longitudinal study were analysed for species in Kasetsart University. DNA of Anopheles specimens was extracted according to procedures of Linton et al. 2001 and Manguin et al. 2002 [48, 49]. Molecular identifications were performed using the AS-PCR assay of Garros et al. 2004 with primers specific for An. minimus s.s. and An. harrisoni for the An. minimus complex, the AS-PCR assay of Walton et al. 2007 with primers specific for An. maculatus s.s. and An. sawadwongporni for the An. maculatus complex and the AS-PCR assay of Walton et al. 1999 and Audtho et al. 1995 with the specific primers for An. dirus s.s., An. cracens, An. scanloni, An. baimaii and An. nemophilous for the An. dirus complex [50-53].
Molecular data, dengue vector viral identification
No plasmodium detections were conducted on malaria vectors, due to the absence of the disease in our study areas. All collected Ae. albopictus samples were screened for arboviral sequences. Additionally all collected Armigeres kesseli and Ar. subalbatus mosquitoes were screened for arboviral sequences.
The abdomen, wings and legs of samples were separated from the thorax and female and male samples pooled separately in 1-10 samples. RNA was extracted using the NucleoSpinR8 Virus, Ref: 740 643.5, extraction kit. The RNA of our samples were amplified using specific primers with RT-PCR and screened for the alphavirus and flavivirus genus sequence using agarose gel electrophoresis. The external set of primers Alpha1- KYT CYT CIG TRT GYT TIG TIC CIG G, Alpha1+ GAY GCI TAY YTI GAY ATG GTI GAI GG was used for reverse transcription and external amplification. The internal primers Alpha 2- GCR AAI ARI GCI GCY TYI GGI CC, Alpha 2+ GIA AYT GYA AYG TIA CIC ARA TG were used for the nested PCR [54]. The external set of primers Flavi1- TCC CAI CCI GCI RTR TCR TCI GC, Flavi1+ GAY TYI GGI TGY GGI IGI GGI RGI TGG was used for reverse transcription and external amplification. The internal primers Flavi 2- CCA RTG ITC YKY RTT IAI RAA ICC, Flavi2+ YGY RTI YTY AWC AYS ATG GC were used for the nested PCR [55].
Results January 2015 – December 2015
Longitudinal study on mosquito ecology in rubber plantation
Analysis of sampling efficiency was made using generalized estimating equations using a negative binomial model for count data with a log-link function (IBM SPSS statistics, ver. 20). Species diversity was compared using the Simpson’s index of diversity (1-D) with the result representing the diversity from 0 (no diversity) to 1 (infinite diversity).
During 3,888 collection hours in each habitat over nine months survey 24,927 female mosquitoes 8.7% were collected in villages (2,164/24,927), 21.4% in immature rubber plantations (5,323/24,927), 14.6% in mature rubber plantations (3,651/24,927) and 55.3% in secondary forest (13,789/24,927) 116 species were collected, including 62 species that have not been recorded in Lao PDR before. 1,249 male mosquitoes were collected of which 71.2% (889/1,249) was Ae. albopictus. 13 female and 9 male mosquitoes could not be identified. More than 60% (9,395/15,552) of the sampling occasions yielded no mosquitoes (80%, 3,111/3,888 in village; 59.2%, 2,300/3,888 in immature; 64.7%, 2,514/3,888 in mature; 37.8%, 1,470/3,888 in forest). In the villages 62 mosquito species were collected with a Simpson’s index of diversity of 0.864 (95% CI 0.855-0.873). This diversity index was higher than for immature rubber plantations where 79 species were collected (1-D= 0.843 with 95% CI 0.838-848) and mature rubber plantations where 72 species were collected (1-D= 0.816 with 95% CI 0.806-0.825). The secondary forest showed similar species diversity to the villages with 89 species found (1-D= 0.853 with 95% CI 0.850-0.856).
The species distribution was quite similar between the tree habitats (immature rubber plantation, mature rubber plantation and secondary forest) during both the rainy and dry season (Table 3, 4) with a high number of Aedes mosquitoes collected in the rainy season and a high number of Culex mosquitoes collected in the dry season. However all tree habitats different considerably with the species distribution of the village where the Culex mosquitoes were dominant during the rainy season and Anopheles mosquitoes during the dry season. In the mature rubber plantations 36.5% (1,331/3,651) of female mosquitoes were Ae. albopictus, a vector of dengue. Furthermore 2.5% (93/3651) of female mosquitoes were the malaria vectors An. minimus s.l., An. maculatus s.l., An. dirus s.l. and An. barbirostris. About 12% (440/3,651) of female mosquitoes were Cx. vishnui (vector of Japanese encephalitis).
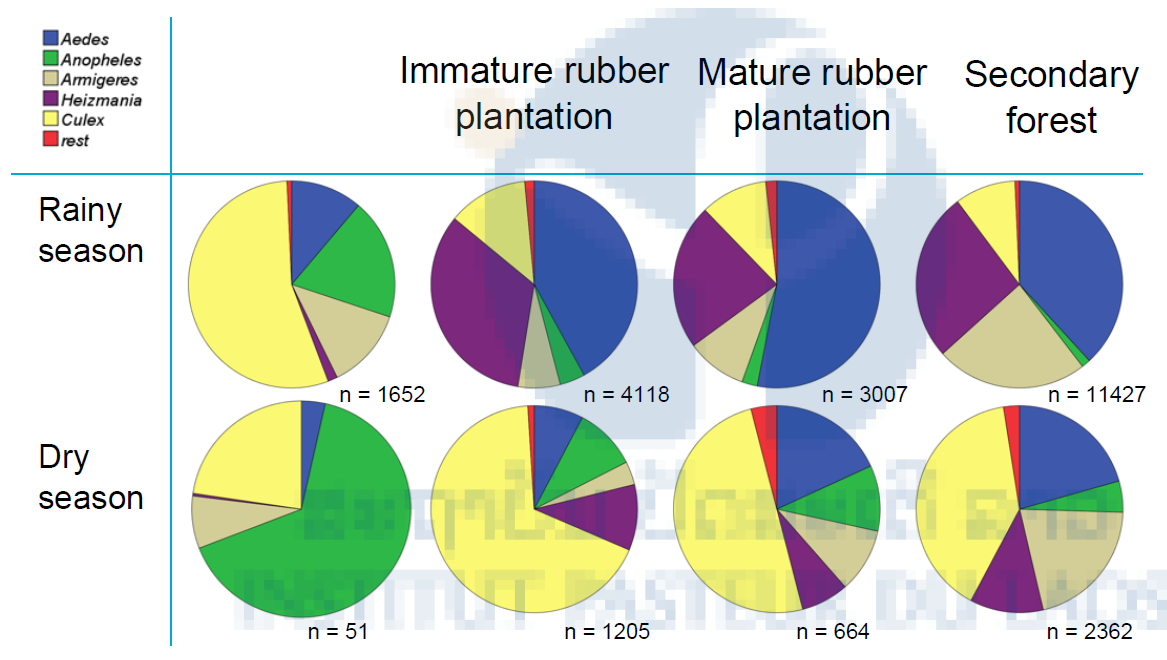
Table 3: Mosquito species distribution in the village, immature rubber plantation, mature rubber plantation and secondary forest during the rainy season from May to October and the dry season from November to April
Aedes mosquitoes were most abundant, of which 73% (6305/8590) was Ae. albopictus mosquitoes. Aedes mosquitoes were collected six times more in the rainy seasons (May to October) than in the dry season with rainfall related to higher number of Aedes mosquitoes. Compared to the villages exposure to Aedes mosquitoes was almost nine times higher in immature rubber plantations (P<0.0001), eight times higher in the mature rubber plantations (P<0.0001) and almost 25 times higher in the forest (P<0.0001) (Table 4).
There was low Anopheles activity throughout the study period for all habitats with 50% more collected during the dry season compared to the wet season. Anopheles species were host-seeking from 18.00-23.00H with low activity from 0.00-05.00H. In all tree habitats two to three times fewer Anopheles mosquitoes were collected than in the villages (all P<0.0001) (Table 4).
Armigeres mosquitoes were collected most frequently in the rainy season with two host-seeking behaviour peaks at 6.00-7.00H and 18.00H. Almost 65% (2,621/4,124) of collected Armigeres mosquitoes were Ar. kesseli mosquitoes. Higher number of Armigeres mosquitoes were collected in the tree habitats compared to the villages with 13 times more Armigeres mosquitoes collected in the forest (P<0.0001) (Table 4).
Most Heizmannia mosquitoes were collected during the rainy season with host-seeking behaviour throughout the day. Only few Heizmannia mosquitoes were collected in the villages with 50 times more collected in the immature rubber plantations (P<0.0001), almost 25 times more in the mature rubber plantations (P<0.0001) and 119 times more in the secondary forest (P<0.0001) (Table 4).
Culex mosquito collection peaks were present both in the rainy and dry seasons. During the rainy season Culex numbers were most abundant in the villages whilst in the tree habitats Culex mosquitoes were most abundant in the dry season. Highest host-seeking behaviour was during the evening with cooler temperatures increasing host-seeking behaviour of Culex mosquitoes. In the villages 1.6 times more Culex mosquitoes were collected than in the mature rubber plantations (P<0.0001). However 1.13 times less Culex mosquitoes were collected than in the immature rubber plantations (P=0.031) and more than two times less than in the secondary forest (P<0.0001) (Table 4).
Most Heizmannia mosquitoes were collected during the rainy season with host-seeking behaviour throughout the day. Only few Heizmannia mosquitoes were collected in the villages with 50 times more collected in the immature rubber plantations (P<0.0001), almost 25 times more in the mature rubber plantations (P<0.0001) and 119 times more in the secondary forest (P<0.0001) (Table 4).
Culex mosquito collection peaks were present both in the rainy and dry seasons. During the rainy season Culex numbers were most abundant in the villages whilst in the tree habitats Culex mosquitoes were most abundant in the dry season. Highest host-seeking behaviour was during the evening with cooler temperatures increasing host-seeking behaviour of Culex mosquitoes. In the villages 1.6 times more Culex mosquitoes were collected than in the mature rubber plantations (P<0.0001). However 1.13 times less Culex mosquitoes were collected than in the immature rubber plantations (P=0.031) and more than two times less than in the secondary forest (P<0.0001) (Table 4).
Table 4: Mean catch size of female mosquitoes per person-hour for village compared to immature rubber plantation, mature rubber plantatio n and secondary forest with odds ratio (OR) and 95% confidence interval (CI) *significantly different, P<0.05
Parity study
During the 42 nights of collection 1,326 vector mosquitoes were collected of which 1,263 were dissected successfully, including 1,171 Ae. albopictus mosquitoes and 92 Anopheles mosquitoes (82 malaria vectors). Unfortunately number of Anopheles malaria vector mosquitoes were very low throughout the collection period with in mature rubber plantations only 10 collected (Table 5). In general the parity rate was high with long living vector mosquitoes present in all habitats.
Table 5: The parity rate of the dengue vector Ae. albopictus and malaria vectors in the village, immature rubber plantation, mature rubber plantation and secondary forest
Vectorial capacity
The vectorial capacity for malaria disease was calculated for Plasmodium falciparum and Plasmodium vivax parasites separately for each habitat for the three most common and important primary vector collected in our study areas; An. maculatus s.l., An. minimus s.l. and An. dirus s.l. All habitats show high vectorial capacity during the dry and wet season. It seems that An. minimus is the main vector for malaria transmission in the different habitats during the dry season, which shifts to An. maculatus during the wet season. An. dirus is not an important malaria vector in our study sites. Similar vectorial capacity can be found for both parasite species, with a slightly higher R0 for P. vivax. The vectorial capacity of dengue was calculated using the primary vector Ae. albopictus. The vectorial capacity in the secondary forest was highest, followed by the mature rubber plantations.
In the next few months the vectorial capacities will be related to the human behavior of the rubber plantation workers and the villagers to understand the risk of infection in each habitat.
Table 6: The vectorial capacity of P. falciparum in the forest, mature rubber plantation, immature rubber plantation and village habitats was calculated for dry and wet season using the vectors An. maculatus s.l., An. minimus s.l. and An dirus s.l.
Table 7: The vectorial capacity of P. vivax in the forest, mature rubber plantation, immature rubber plantation and village habitats was calculated for dry and wet season using the vectors An. maculatus s.l., An. minimus s.l. and An dirus s.l.
Table 8: The vectorial capacity of dengue in the forest, mature rubber plantation, immature rubber plantation and village habitats was calculated for dry and wet season with the primary vector Ae. albopictus
Larval survey
During the five monthly surveys in immature rubber plantations, mature rubber plantations and villages 1,384 waterbodies were surveyed. A total of 52% (725/1,384) of waterbodies surveys were positive for mosquito larvae. Interestingly we found Culex, Armigeres and Aedes mosquito larvae in the latex collection cups, even when latex was still present. Aedes larvae were furthermore found breeding in latex collection cups, banana plant axils, leaves of banana plants and in garbage discarded by rubber workers, including tires and coconut shells. We found Anopheles mosquitoes breeding in road side puddles and in small puddles next to the streams (Figure 5).
Figure 5: Larval breeding sites, including old discarded tires, leaves of banana plants and latex collection cups
Molecular data, malaria vector species identification
Unfortunately none of the subspecies of An. dirus could be identified due to problems with the PCR at Kasetsart University. However for An. minimus and An. maculatus the following species were identified.
An. minimus complex
+ Anopheles minimus 86 samples
+ Anopheles aconitus 57 samples
+ Anopheles varuna 1 sample
An. maculatus complex
+ Anopheles maculatus 180 samples
+ Anopheles pseudowillmori 35 samples
+ Anopheles sawadwongporni 9 samples
+ Anopheles dravidicas 10 samples
Molecular data, dengue vector viral identification
We tried to identify the vector of dengue in our study area where during our study period from July 2013-June 2014, 199 dengue cases were reported in study district Xieng Ngeun and 11 cases in Nane district. All samples of Aedes albopictus were screened for the presence of the alphavirus and flavivirus in pools of 1 to 10 mosquitoes. A total of 1,252 Ae. albopictus pools were tested for alphavirus and flavivirus sequences. None of the pools displayed amplicon of expected size for alphavirus. For flavivirus, 36 positive pools were identified. The positive pools of flavivirus were from both male and female mosquito pools and mosquitoes collected from mature rubber plantations, immature rubber plantations and secondary forest. Further analysis of these positive pools has not given any information on the specific flavivirus present in the samples.
The possible secondary dengue vector Armigeres kesseli /Ar. subalbatus was also analysed for alpha and flavivirus presence. Of the 510 pools tested, none displayed amplicons of expected size.
National communication
The emphasis of this ECOMORE project is on the communication with the stakeholders within Lao PDR. We have therefore organized multiple village meetings in our study areas throughout 2015, with our final one conducted in September 2015. These village meetings were organized to share our results and recommendations and have close relations with the villages we work with. Furthermore an ECOMORE National Stakeholders meeting was organized in Vientiane Capital to discuss our findings and recommendations with the government and other important stakeholders within the country. Moreover we participated in the Strategic Plan for Health meeting in Luang Prabang province and shared our results with the Department of Communicable Disease Control, Ministry of Health of Lao PDR (Figure 6).
Figure 6: Images of our village meeting, National Stakeholders meeting and participation in the Strategic Plan for Health meeting
Currently we are also in the process of including a health Chapter in the government Rubber Information book (both Lao and English language). This chapter focussed on work-related injuries (risks and prevention), environment-related injuries (risks and prevention) and pathogenic diseases (risks and prevention indoor, outdoor and larval source management). The inclusion of this chapter in the information book will hopefully make rubber industry stakeholders more aware of the risks related to working in rubber plantations and how to prevent these.
Interational communication
Apart from communication of our project and results within Lao PDR we attended several meetings to share our results. We gave an oral presentation at the 3rd GRF One Health Summit 2015 in Davos, Switzerland. Furthermore we presented a poster at the International Scientific Symposium – Institut Pasteur International Network 2015 in Paris, France. We also presented our work at the Vientiane International symposium on ethics in research for international development (Figure 7). Additionally we published our first scientific paper in the open source journal of PloS One, named ‘The Human-Baited Double Net Trap: an Alternative to Human Landing Catches for Collecting Outdoor Biting Mosquitoes in Lao PDR’.
Figure 7: Images of the GRF One health summit presentation, the poster presentation at the International Scientific Symposium of Pasteur Paris and the presentation at the Vientiane International symposium on ethics
Training provision
The ECOMORE project not only conducted research, we also trained an entomologist from Myanmar, Mr. Saw Mitchell, at our institute in Lao PDR for 6 weeks. He received basic training in entomology, including field training to collect mosquito larvae, adult mosquito identification, training in different collection techniques (CDC light trap, BG sentinel, Human-baited Double Net trap, Human Landing Catches) and how to work in the entomological colony room (Figure 8).
Figure 8: Images of the training of Mr. Saw Mitchell; the team, the fieldwork and the laboratory work
Opportunities in Africa
The ECOMORE project has been such a success that we have now started working together with Institut Pasteur de Côte d’Ivoire to do a mirror study in their country where malaria is endemic. This south-south cooperation between the two institutes will be a great way for two developing countries to share knowledge and experience. Especially for the Lao team to share their knowledge and experience from the ECOMORE project will be invaluable for the success of the Ivorian project. The Lao team has visited the project in Côte d’Ivoire as has the team from Côte d’Ivoire visited the field sites and laboratory in Lao PDR (Figure 9).
Figure 9: Images of the two teams working together; in the field and in the laboratory
Future activities
The ECOMORE project will come to an end in July 2016. Before the end of the project we will focus on finishing the rubber information book. We will work closely together with NAFRI to ensure the updated book, including our health chapter, will be available for the rubber industry stakeholders before the end of our project. We will finish the analysis of the longitudinal study and larval survey and publish our results in scientific journals. Most importantly we will conduct a study in the field to compare different commercially available methods in which rubber workers can protect themselves from mosquito bites whiles tapping latex. The results from this study will be communicated to the interested stakeholders to ensure a proper recommendation can be made concerning protection methods in the plantations.
References
1. Coker RJ, Hunter BM, Rudge JW, Liverani M, Hanvoravongchai P: Emerging infectious diseases in southeast Asia: regional challenges to control. Lancet 2011, 377:599-609.
2. Newhouse VP, Chamberlain, R.W., Johnston, J.G., Sudia, W.D. : Use of dry ice to increase mosquito catches of the CDC miniature light trap. Mosquito News 1966, 26:30-35 3.
3. Dengue and severe dengue Fact sheet N°117 [http://www.who.int/mediacentre/factsheets/fs117/en/]
4. Japanese encephalitis, Fact sheet No 386 [http://www.searo.who.int/thailand/factsheets/fs0011/en/]
5. Kilpatrick AM, SE R: Drivers, dynamics, and control of emerging vector-borne zoonotic diseases. Lancet 2012, 380(9857):1946-1955.
6. WHO: Agricultural development and vector-borne diseases: Training and information materials on vector biology and control. Geneva: WHO, FAO, UNEP, UNCHS PEEM; 1996.
7. Woolhouse ME, S. G-S: Host range and emerging and reemerging pathogens. Emerging infectious diseases 2005, 11(12):1842-1847.
8. Petney T, Sithithaworn P, Satrawaha R, Grundy-Warr C, Andrews R, Wang Y-C, Feng C-C: Potential Malaria Reemergence, Northeastern Thailand. Emerging infectious diseases 2009, 15(8).
9. Jones KE, Patel NG, Levy MA, Storeygard A, Balk D, Gittleman JL, Daszak P: Global trends in emerging infectious diseases. Nature 2008, 451:990-993.
10. Waerden G: Chinese economic boom. In: The Guardian 2010.
11. Worldbank: World data bank. 2012.
12. PWC pwc: GDP projections from PwC: how China, India and Brazil will overtake the West by 2050. In: The Guardian 2011.
13. IMF IMF: World economic outlook: Rebalancing Growth. Washington DC 2010.
14. PDO PsDO: China’s development benefited the surrounding countries. In: PDO, Peoples Daily Online 2010.
15. International Rubber Study Group: Rubber industry report. Singapore: IRSG; 2012.
16. Chinese Auto Sales Set New World Record of 18 Million Units in 2010 [http://chinaautoweb.com/2011/01/chinese-auto-sales-set-new-world-record-of-18-million-units-in-2010/]
17. Sumernet: Discussion paper: rubber investment and market linkages in Lao PDR: approaches of sustainability. Edited by Network SMR2009.
18. Rubber daily price [http://www.indexmundi.com/commodities/?commodity=rubber&months=180]
19. Food and Agriculture Organization: Annual producer price indices for natural rubber. Edited by STAT F. Rome: FAO; 2013.
20. International rubber price [http://rubberboard.org.in/Internationalrubberprice.asp?
month=5&year=2011&submitmonth=Go]
21. International Rubber Study Group: Statistical summary of world rubber situation. Statistical Rubber Bulletin 2014, April – June
22. Vientiane times reporters: Farmers fear rubber price may not bounce back. In: Vientiane Times 2014.
23. Phuc LGT: Rubber based farming system applicable for immature smallholdings in highland central coastal region of Vietnam. Rubber research Institute of Vietnam; 2006.
24. Food and Agriculture Organization: Global forest resources assessment 2010. Rome: FAO; 2010.
25. Summary of rubber data [www.Rubberthai.com ]
26. Thai Rubber Association: 60th anniversary of the Thai rubber association. Bangkok: Ministry of Agriculture and Cooperatives; 2011.
27. Li Z, Fox JM: Mapping rubber tree growth in mainland Southeast Asia using time-series MODIS 250 m NDVI and statistical data. Appl Geogr 2012, 32:420-432.
28. Daily price [http://www.anrpc.org/html/daily_prices.aspx]
29. Monthly price [http://www.sunsirs.com/uk/sdetail-month–.html]
30. Krishnakumar P: Natural rubber output grows 3% in December. In: The Economic Times 2013. 31. post B: IRC: Rubber stockpiles ‘low, declining’. In: Bangkok Post, 10 February edn. Bangkok2014.
32. Huileng T: China Soybean, Rubber Importers Renege on Deals; Canceled Contracts Add to Evidence Showing Rising Financial Stress in the Country. In: Wall Street Journal. New York2014.
33. Rubber prices in a skid [http://globalrubbermarkets.com/18644/rubber-prices-skid.html]
34. Fredrickson T: Rubber protest turns violent. In: The Bangkok Post 2013.
35. Times NY: In Thailand, Rubber Price Plunge Has Political Cost. In: New York Times. New York2013. 36. NAFRI NaaFRILP: Review of rubber plantations. Vientiane, Lao PDR2011.
37. Dondero TJJ, Menon VV: Clinical epidemiology of filariasis due to Brugia malayi on a rubber estate in west Malaysia. Southeast Asian J Trop Med Public Health 1972, 3(3):355-365. 38. Singh J, Tham AS: Case history on malaria vector control through the application of environmental management in Malaysia. vol. 154776. Geneva, Switserland: WHO; 1988.
39. Singhasivanon P, Thimasarn K, Yimsamran S, Linthicum K, Nualchawee K, Dawreang D, Kongrod S, Premmanisakul N, Maneeboonyang W, Salazar N: Malaria in tree crop plantations in south-eastern and western provinces of Thailand. Southeast Asian Journal of Tropical Medicine and Public Health 1999, 30 (3):399-404.
40. Yasuoka J, Levins R: Impact of deforestation and agricultural development on anopheline ecology and malaria epidemiology. American society of tropical medicine and hygiene 2007, 76(3):450-460.
41. Watson M: The prevention of malaria in the Federated Malay States, a record of 20 years progress. New York: E.P. Dutton and company; 1921.
42. Jomon KV, Valamparampil TT: Medically important mosquitoes in the rubber plantation belt of central kerala, india. Southeast Asian J Trop Med Public Health 2014, 45(4):796.
43. Sumodan PK: Potential of rubber plantations as breeding source for Aedes albopictus in Kerala, India. Dengue Bulletin 2003, 27:197-198.
44. Sumodan PK: Species diversity of mosquito breeding in rubber plantations of Kerala, India. Journal of the American Mosquito Control Association 2012, 28(2):114-115.
45. Rosenberg R, Andre RG, Somchit L: Highly efficient dry season transmission of malaria in Thailand. Trans R Soc Trop Med Hyg 1990, 84(1):22-28.
46. Obsomer V, Defourny P, Coosemans M: The Anopheles dirus complex: spatial distribution and environmental drivers. Malaria Journal 2007, 6(26).
47. Detinova TS: Determination of the physiological age of female Anopheles from the changes of the tracheal system of the ovaries. Meditsinskaia parazitologiia i parazitarnye bolezni 1945, 14:45-49.
48. Linton YM, Harbach RE, Chang Moh S, Anthony TG, Matusop A: Morphological and molecular identity of Anopheles (Cellia) sundaicus (Diptera: Culicidae), the nominotypical member of a malaria vector species complex in Southeast Asia Systematic Entomology 2001, 36:357-366.
49. Manguin S, Kengne P, Sonnier L, Harbach RE, Baimai V, Trung HD, Coosemans M: SCAR markers and multiplex PCR-based identification of isomorphic species in the Anopheles dirus complex in Southeast Asia. Medical and Veterinary Entomology 2002, 16:46–54.
50. Garros C, Koekemoer LL, Coetzee M, Coosemans M, Manguin S: A single multiplex assay to identify major malaria vectors within the African Anopheles funestus and the Oriental An. minimus groups. American Journal of Tropical Medicine and Hygiene 2004, 70:583-590.
51. Walton C, Somboon P, O’Loughlin SM, Zhang S, Harbach RE, Linton YM, Chen B, Nolan K, Duong S, Fong MY et al: Genetic diversity and molecular identification of mosquito species in the Anopheles maculatus group using the ITS2 region of rDNA. Infections, Genetics and Evolution 2007, 7:93–102.
52. Walton C, Handley JM, Kuvangkadilok C, Collins FH, Harbach RE, Baimai V, Butlin RK: Identification of five species of the Anopheles dirus complex from Thailand, using allele-specific polymerase chain reaction. Medical and Veterinary Entomology 1999, 13:24-32.
53. Audtho M, Tassanakajon A, Boonsaeng V, Tpiankijagum S, Panyim S: Simple nonradioactive DNA hybridization method for identification of sibling species of Anopheles dirus (Diptera: Culicidae) complex. Journal of Medical Entomology 1995, 32:107-111.
54. Sánchez-Seco MP, Rosario D, Quiroz E, Guzmán G, Tenorio A: A generic nested-RT-PCR followed by sequencing for detection and identification of members of the alphavirus genus. Journal of Virological Methods 2001, 95(1–2):153-161.
55. Sánchez-Seco MP, Rosario D, Domingo C, Hernández L, Valdés K, Guzmán MG, Tenorio A: Generic RT-nested-PCR for detection of flaviviruses using degenerated primers and internal control followed by sequencing for specific identification. Journal of Virological Methods 2005, 126(1–2):101-109.